Fast control methods enable record-setting fidelity in superconducting qubit
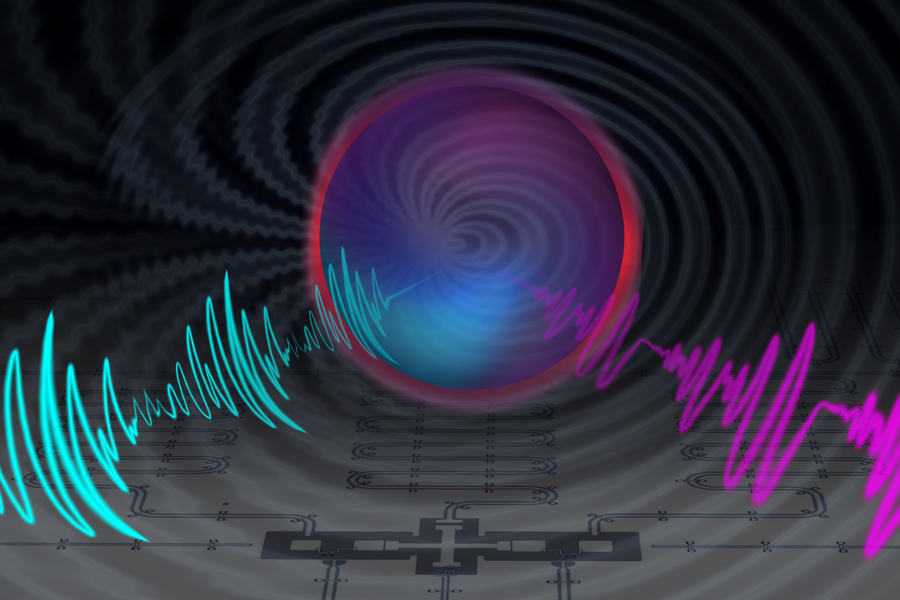
Quantum computing, which leverages the principles of quantum mechanics to process information using quantum bits (qubits), holds the potential to solve highly complex problems much faster than traditional computers. However, a significant challenge in scaling quantum systems lies in the sensitivity of qubits to noise and control imperfections, which introduce errors and limit the effectiveness of quantum operations. Addressing this, researchers at MIT and around the world are continuously innovating to enhance qubit performance.
In recent research, a team at MIT's Department of Physics, Research Laboratory of Electronics (RLE), and Department of Electrical Engineering and Computer Science (EECS) achieved a groundbreaking milestone using a superconducting qubit called fluxonium. They developed two novel control methods, setting a world record for single-qubit fidelity at 99.998 percent. This achievement builds on earlier work by Leon Ding, a former MIT researcher, who demonstrated a two-qubit gate fidelity of 99.92 percent.
The study, recently published in PRX Quantum, was led by senior authors David Rower, a PhD graduate from MIT and current research scientist at Google Quantum AI, Leon Ding, now heading the Calibration team at Atlantic Quantum, and William D. Oliver, a professor at MIT and director of the Center for Quantum Engineering.
Tackling Decoherence and Counter-Rotating Errors
Decoherence, the process through which qubits lose their quantum information, is a major hurdle for quantum computing platforms like superconducting qubits. High gate fidelities are critical for implementing quantum error correction, which enables sustained quantum computation.
To counter the impact of decoherence, MIT researchers are focusing on making quantum gates faster. However, faster gates introduce counter-rotating errors, which occur due to the way electromagnetic waves control qubits. These errors, particularly problematic for low-frequency qubits like fluxonium, can limit gate fidelity.
To address this, the team initially experimented with circularly polarized microwave drives, analogous to circularly polarized light, to minimize counter-rotating errors. Although promising, the fidelities achieved with this approach fell short of theoretical expectations.
The breakthrough came with the introduction of "commensurate pulses," a simple yet effective technique involving the timing of pulses at specific intervals relative to the qubit frequency. By synchronizing pulse timing, counter-rotating errors became consistent and correctable, aligning seamlessly with standard Rabi gate calibrations. This method proved to be universally applicable to any qubit affected by counter-rotating errors.
The Potential of Fluxonium
Fluxonium, a type of superconducting qubit incorporating a capacitor, Josephson junction, and a "superinductor," is designed to resist environmental noise, enabling higher accuracy during logical operations. While its lower frequency typically results in longer gate times, the research demonstrated that fluxonium can achieve fast, high-fidelity gates, making it a strong candidate for advanced quantum computing.
The team’s findings revealed effective ways to overcome the limitations of counter-rotating dynamics, paving the way for faster and more precise quantum gates. These advancements highlight fluxonium's suitability for both fundamental physics research and engineering applications in quantum computing.
A Collaborative Approach
The success of this project lies in the intersection of physics and engineering. By combining non-adiabatic control techniques with advanced qubit design, the researchers achieved higher fidelity and performance. Their work also extends the understanding of counter-rotating dynamics, which are often overlooked in superconducting quantum computing due to the common use of the rotating-wave approximation.
This research offers a platform-independent solution to counter-rotating errors without requiring additional calibration, providing a straightforward method to enhance control fidelity across various quantum computing platforms.
In the context of recent advancements, such as Google’s Willow quantum chip demonstrating error correction beyond threshold, this work pushes the boundaries of performance even further. High-fidelity qubits like those demonstrated in this study will reduce the overhead required for implementing fault-tolerant quantum computing.
This research involved contributions from numerous scientists at MIT, RLE, and MIT Lincoln Laboratory and was supported by agencies including the U.S. Army Research Office, the Department of Energy, and the National Science Foundation.